Microsoft’s Majorana One Chip: The Topological Quantum Leap That Could Change the Future of Computing
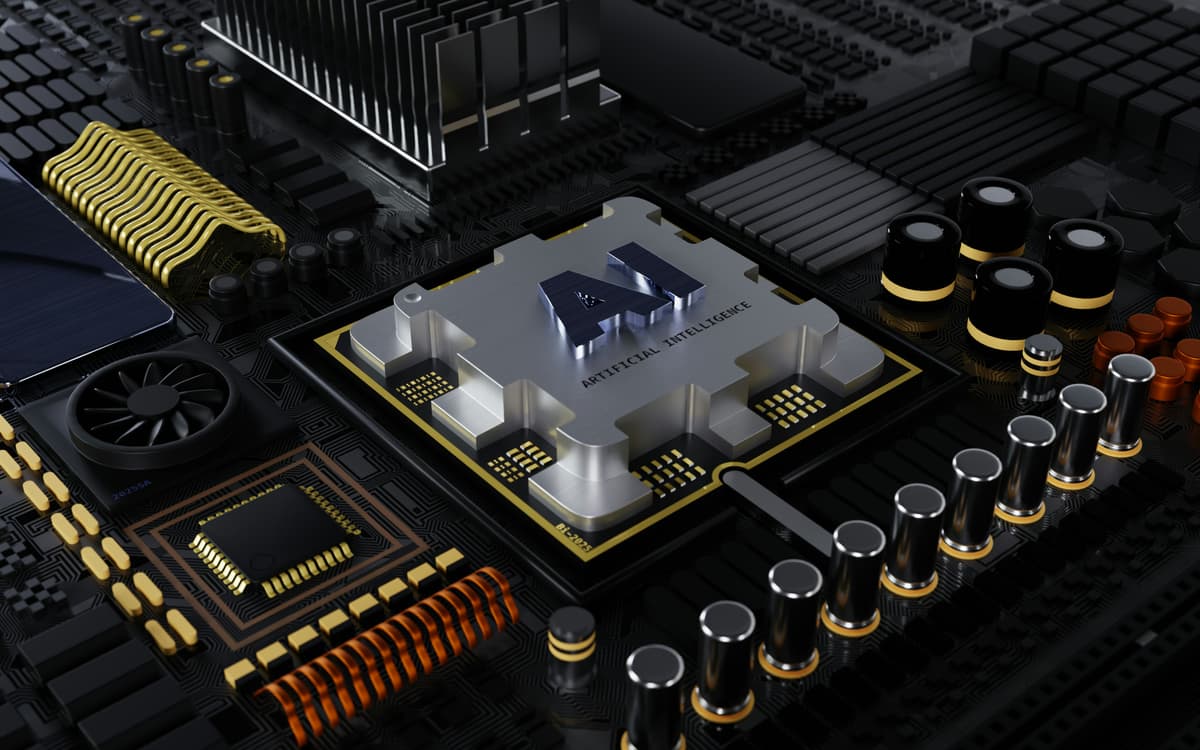
Written by Massa Medi
Microsoft has just ignited the quantum computing world with its unveiling of the Majorana One chip—the planet’s first topological qubit device. Unlike anything before, this chip sidesteps the quantum fragility that has hobbled every quantum system so far, carrying within it promises to transform chemistry, climate science, and computation itself. Here’s an in-depth look at why the Majorana One—and topological quantum computing—might signal a new era, based on exclusive interviews with Microsoft’s key quantum architects.
Not Your Average Quantum Leap: What Makes Majorana One Unique?
In the wild world of quantum computing, innovations usually revolve around the delicate manipulation of atoms, ions, or photons. Microsoft’s Majorana One, however, flips the script. Rather than storing information in localized quantum states susceptible to every stray speck of interference, it encodes quantum information across an entire surface—a topology—making it drastically more resilient to the disruptive “noise” that sabotages traditional quantum systems.
What does that mean? In simplest terms: quantum info gets woven in ways that natural errors struggle to unravel. It’s not about making things merely better—it’s about changing the rules of the quantum game.
“To try and better understand what is exactly going on here...we had to essentially invent a new state of matter.”
As part of this breakthrough, Microsoft granted exclusive access to their core quantum team, intent on explaining not just what the Majorana One means for the future, but also what it doesn’t. And, if you’re like many of us, perhaps questioning whether “Majorana” is even pronounceable by the average mortal.
Why Quantum Computers Break So Easily: The Struggle With Noise
Let’s begin with today’s quantum computers: they promise power to outpace the world’s best supercomputers, offering new frontiers for drug discovery, material science, and tackling optimization problems that stump even the greatest classical machines. But with great power comes... grievous fragility.
Qubits, the building blocks of quantum computing, exist in superpositions—effectively being 0, 1, or somewhere in between. Yet, the act of observing them (even a rogue vibration or stray photon) collapses their quantum state—a process famously known as decoherence. In quantum computers, this wavefunction collapse spells disaster, irreversibly destroying computations mid-flight.
How Do We Protect Qubits?
Quantum machines are wrapped in elaborate shrouds—resembling sci-fi props—constructed to be vacuum-tight, frigidly cold (roughly 50 millikelvin, a whisker above absolute zero), and shielded from electromagnetic interference. Even so, a random, ultra-tiny disturbance can bring a quantum calculation crashing down in an instant.
If you ever wondered, “Can’t we just fix these errors?”—in classical computing, redundancy and error-checking work beautifully. For qubits, things are trickier. The “no cloning theorem” prohibits straightforward copying, so correcting errors involves massive redundancy: hundreds or thousands of delicate physical qubits are wrangled to maintain just a single logical qubit. This scaling nightmare means building a truly useful quantum device would require apparatus the size of a sports stadium.
The Topological Revolution: Let Physics Do The Heavy Lifting
Microsoft’s quantum team, led by innovators like Chetan Naik, is opting for a radical detour. Why not stop fighting noise with brute force, and design hardware where quantum information is naturally immune to it?
Enter topology—the mathematical study of properties unchanged by stretching or deformation. Picture tying a knot in a rope: you can twist or waggle it, but only cutting the rope gets rid of the knot. Likewise, topological quantum computation weaves quantum information into the very fabric of the system, making it robust against everyday disturbances.
“We are saying, hey, first step is get hardware protection built in at the ground level.”
By distributing quantum information so that it’s not localized, these topological qubits become exceptionally resistant to being knocked out of alignment. But to bring this vision to life, scientists needed something even stranger—a quantum object so peculiar, it took nearly a century to find: the Majorana particle.
The Enigmatic Majorana Particle: A Quantum Ghost Story
First described by Ettore Majorana, one of pre-WWII physics’ brightest stars, this particle has a bizarre superpower: it is its own antiparticle. That’s right—while electrons and positrons annihilate each other, a Majorana is its own yin and yang.
Majorana himself vanished mysteriously in 1938, leaving behind theories as enigmatic as his own fate. Scientists spent decades searching for Majorana particles in the wild (particularly among elusive neutrinos), to no avail. The solution came not from cosmic collisions, but from tabletop nanotech.
Building a Topological Qubit From Scratch
In 2012, researchers at Delft University demonstrated that under precise, extraordinarily challenging conditions—using semiconductor nanowires layered with superconductors at frigid temperatures—Majorana-like behaviors magically emerged.
If you imagine a single wire, with an electron’s presence split and distributed across its ends, you have the basic recipe. Add a thin superconducting layer, apply a magnetic field, and you create a state where two “Majorana zero modes” appear at each end: quasi-particles that are, in a sense, each their own antiparticle, each harboring half a quantum state.
Microsoft calls these custom nanowires “topo conductors”—a new, previously unseen state of matter, rivaling the familiar solids, liquids, and gases. This new state allows for the creation of qubits whose information is globally distributed and, crucially, protected.
From Fragile Qubits to Hardware Fault Tolerance
Most quantum information schemes store their states locally, making them vulnerable to random interference. Majorana states are different: the quantum information isn’t localized in one spot. Add—or remove—an electron at one end, and it doesn’t completely destroy the overall state, because the info is smeared over the entire nanowire.
Errors that would obliterate ordinary qubits simply bounce off these topologically protected states, reducing the need for the sprawling, redundant error correction that has kept quantum computers from scaling. Where previous quantum systems have been fixated on quantity (“more qubits!”), Microsoft is betting on quality: if every qubit is resilient by design, you don’t need millions to maintain stability.
Majorana One: Eight Qubits That Change Everything
The result? Microsoft’s Majorana One chip. It features eight proof-of-concept topological qubits—tiny by classical standards, but a revelatory leap for architecture. Detection, control, and readout of these qubits have been achieved for the first time, and as Microsoft’s team notes, this is less about the current count, and more about the roadmap: now that topological fault-tolerance is in hand, scaling up becomes (relatively) a matter of engineering, not new science.
“If reliably engineered quantum computers are only three years away...it begs the question: what are we actually going to do with them?”
Microsoft’s ambition is to leap from 8 to a million stable qubits—a jump that would have sounded impossibly optimistic a decade ago. But when asked, Microsoft’s experts now say it may be “years, not decades,” away, as the challenge shifts from scientific miracles to predictable engineering.
What Will Topological Quantum Computers Do?
So what does all this quantum wizardry actually unlock? The answer, as explained by Nathan Baker (head of Microsoft’s Quantum Applications team), is chemistry—more specifically, chemical reactions at scales and complexities that traditional computers can’t even approximate.
Most important chemical problems ride on the “energy landscape”—the complex array of energy barriers and valleys governing how and whether reactions happen. Accurately simulating these barriers is brutally hard; even small errors mean the difference between a benign environmental byproduct and a forever chemical.
Classical computers choke on reactions involving more than ~24 electron orbitals. Even modeling solutions like ozone’s interaction with chlorine—which netted a Nobel prize—requires approximations that can throw predictions thousands of times off. If quantum computers can render these calculations accurate “first time right,” new drugs, catalysts, or eco-friendly molecules could be designed—to order—radically accelerating innovation.
Quantum Hype vs. Scientific Scrutiny: The Current Controversy
No great leap forward comes without skepticism. Quantum computing, infamously, has been a trail of optimism and setbacks. Microsoft itself saw a major paper retracted in 2021 when independent analysis found flaws in their Majorana claims. Their latest Nature paper, while announcing the Majorana One, does not (as of publication) provide direct, empirical evidence of functioning topological qubits—a fact highlighted by Nature’s editorial team and fiercely debated by quantum physicists worldwide.
Microsoft answers that subsequent, yet-unpublished data (presented at scientific conferences like StationQ and the American Physical Society meeting) shows parity measurements and functional qubit behavior. In other words: the story’s moving fast, perhaps faster than peer-reviewed publications can keep up. But for now, the physics community remains cautiously excited.
“Skepticism is healthy. That’s science. But so is that excitement.”
Even with the controversy, if Microsoft’s topological approach truly yields exponentially more robust qubits, it will be not just a leap for computing, but a transformative turning point for science and civilization at large.
The Human Spirit of Discovery
At its heart, quantum computing’s story is humanity’s story: our urge to look at impossibly complex reality and whisper: “I bet we could do it.” Unlike every other creature on Earth—no matter how clever their tool-using, art-making, or language—only humans have built machines to see the unseeable, to make sense of the universe’s deepest puzzles, and now, perhaps, to outwit the strange probabilities of quantum physics itself.
If you care about science and the future, now is the moment to pay attention—to watch, question, challenge, and celebrate as we push against the limits of what seemed impossible.
Want more on quantum breakthroughs? Follow for future deep-dives and exclusive interviews with Microsoft’s quantum pioneers. If you’re a channel member, full interviews with Zulfi, Chetan, and Nathan will be made available soon.
Like what you read? Leave a comment below, or spread the word. Until next time—stay curious.
Recommended Articles
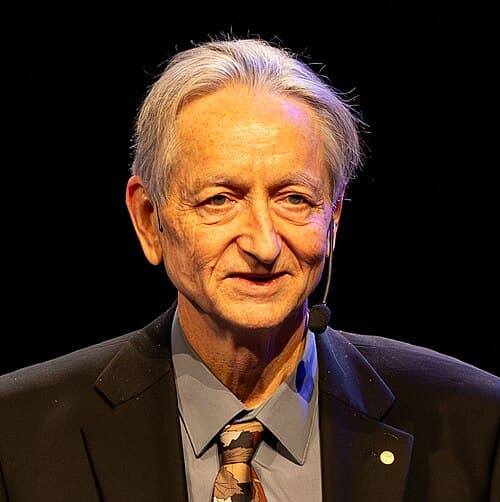
Geoffrey Hinton: The “Godfather of AI” Sounds the Alarm: From Neural Nets to Nobel Prizes and the Uncharted Future of Artificial Intelligence
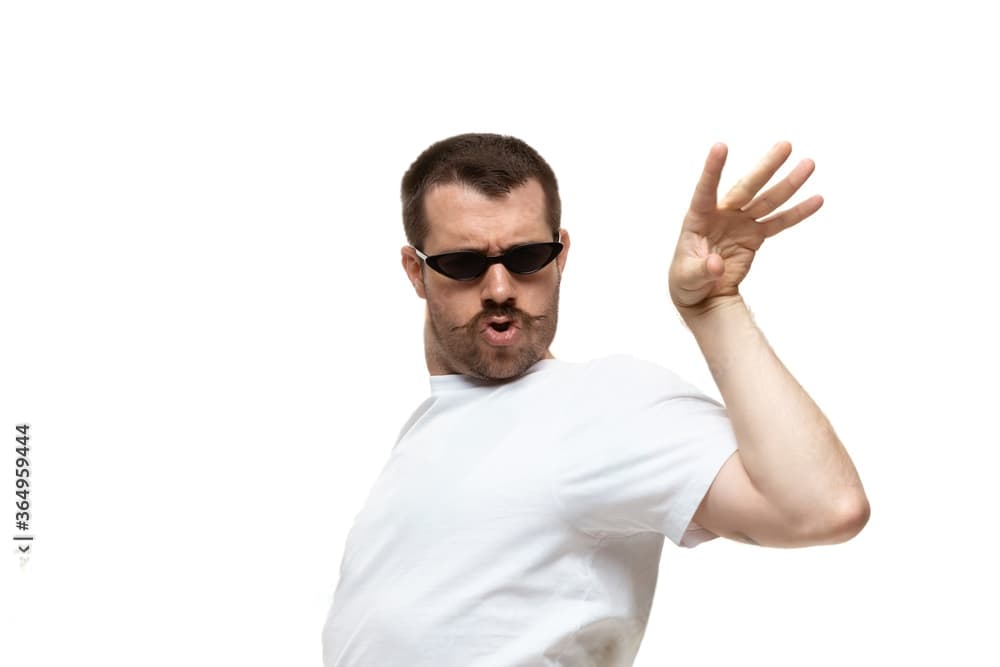
The Rise of Model Context Protocol (MCP): Why Every Developer Is Talking About It
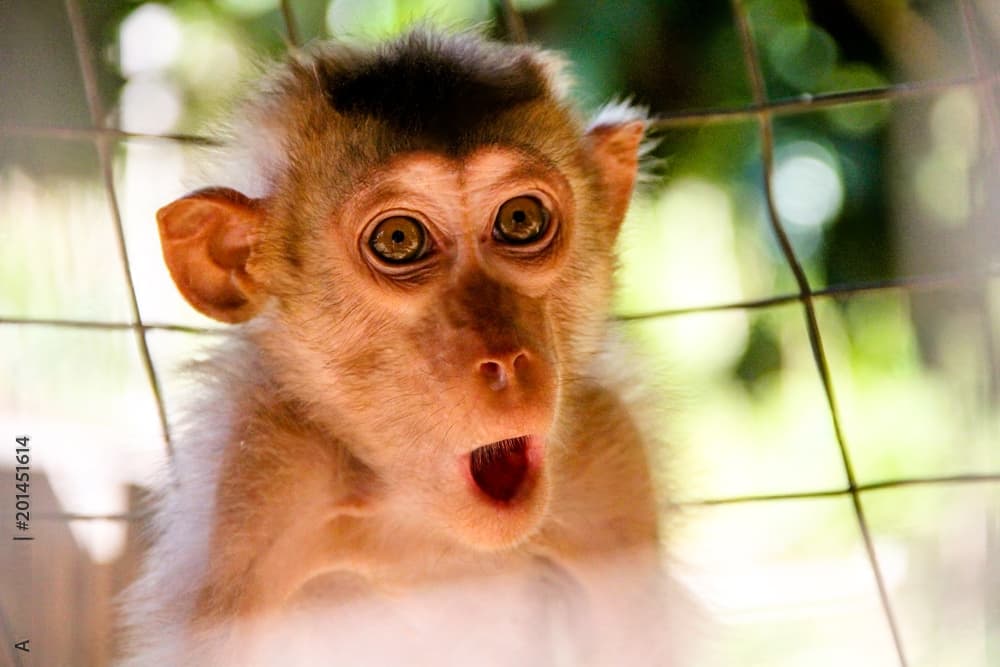
Inside the Magic of Large Language Models: How AI Autocompletes Human Thought
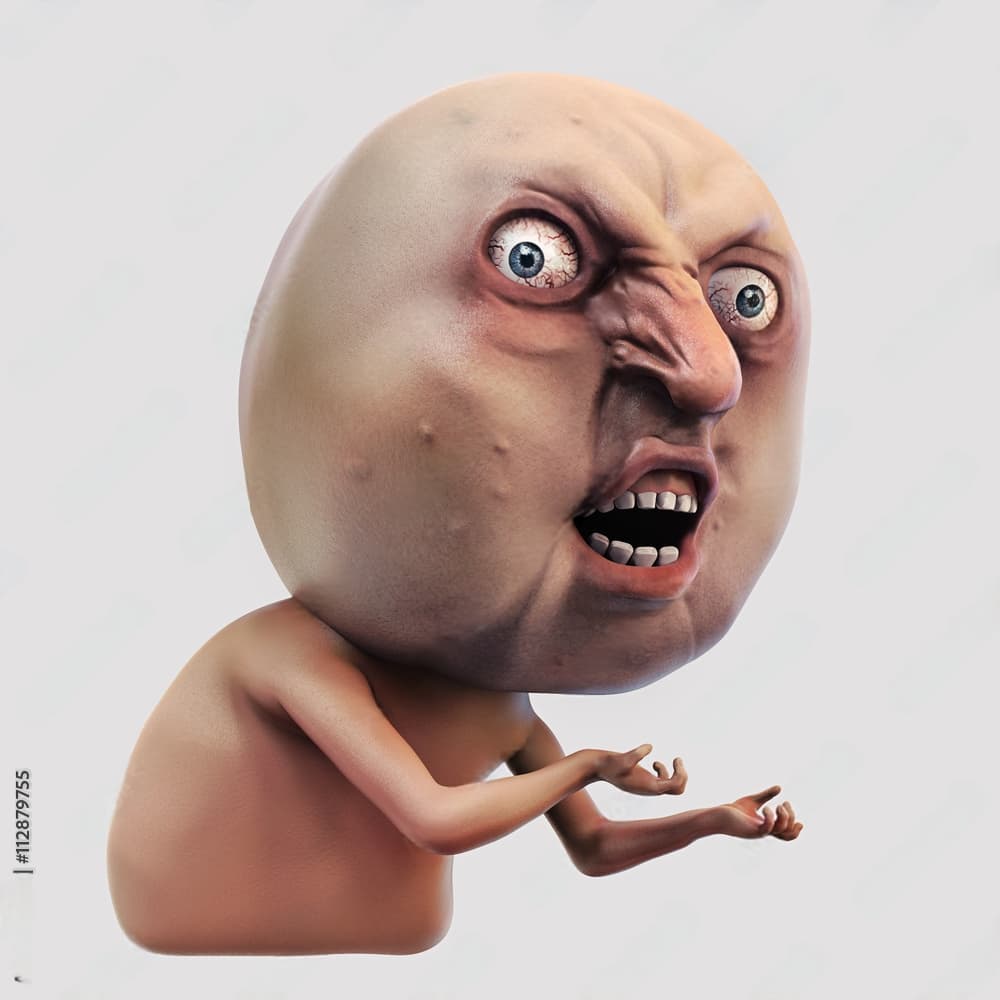
The Evolution of Artificial Intelligence: From Rules to Cosmic Consciousness
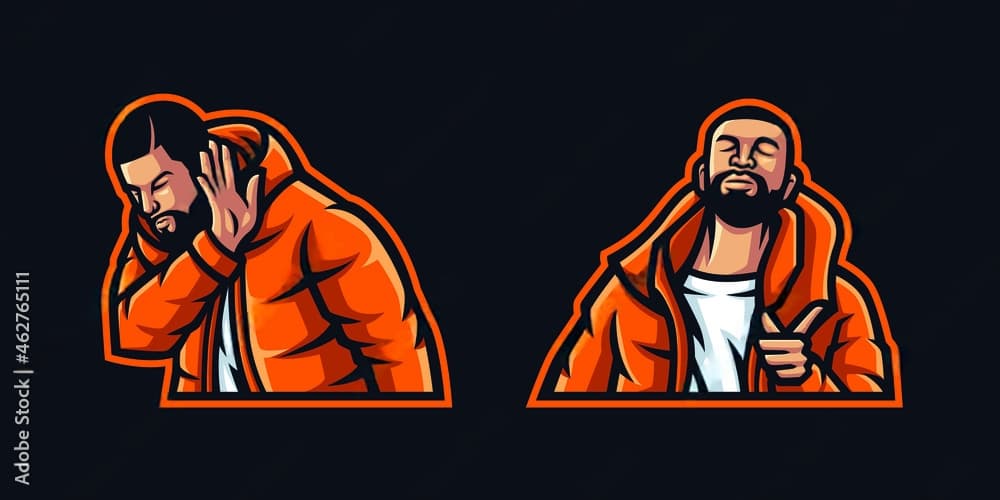
A Hands-On Review of Google’s AI Essentials Course: 5 Key Lessons, Honest Pros & Cons, and Is the Certificate Worth It?
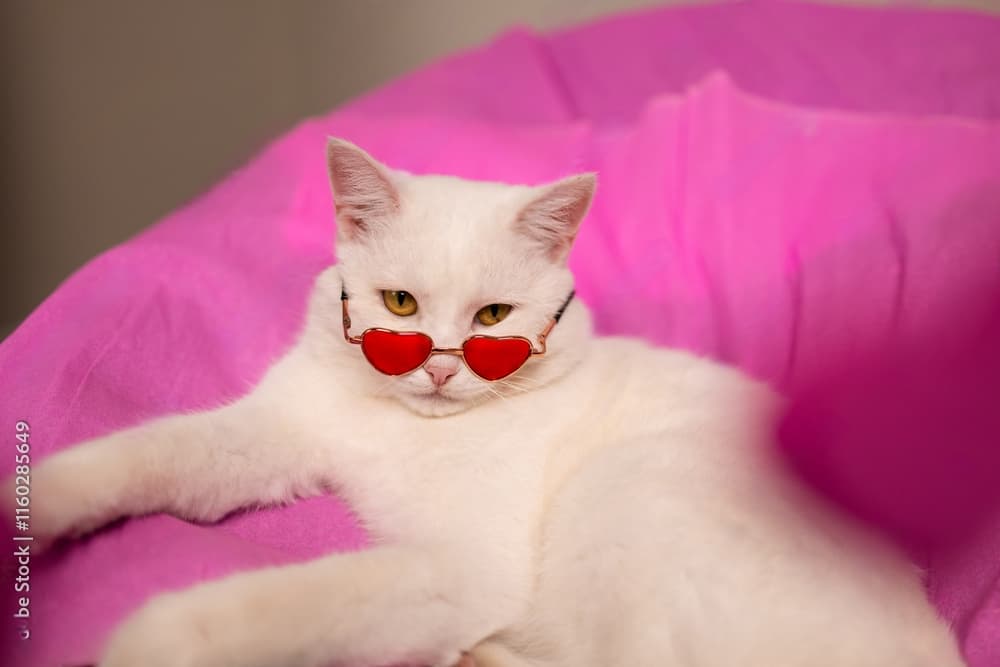
Why Human Connection Still Beats Technology—Even in the AI Era
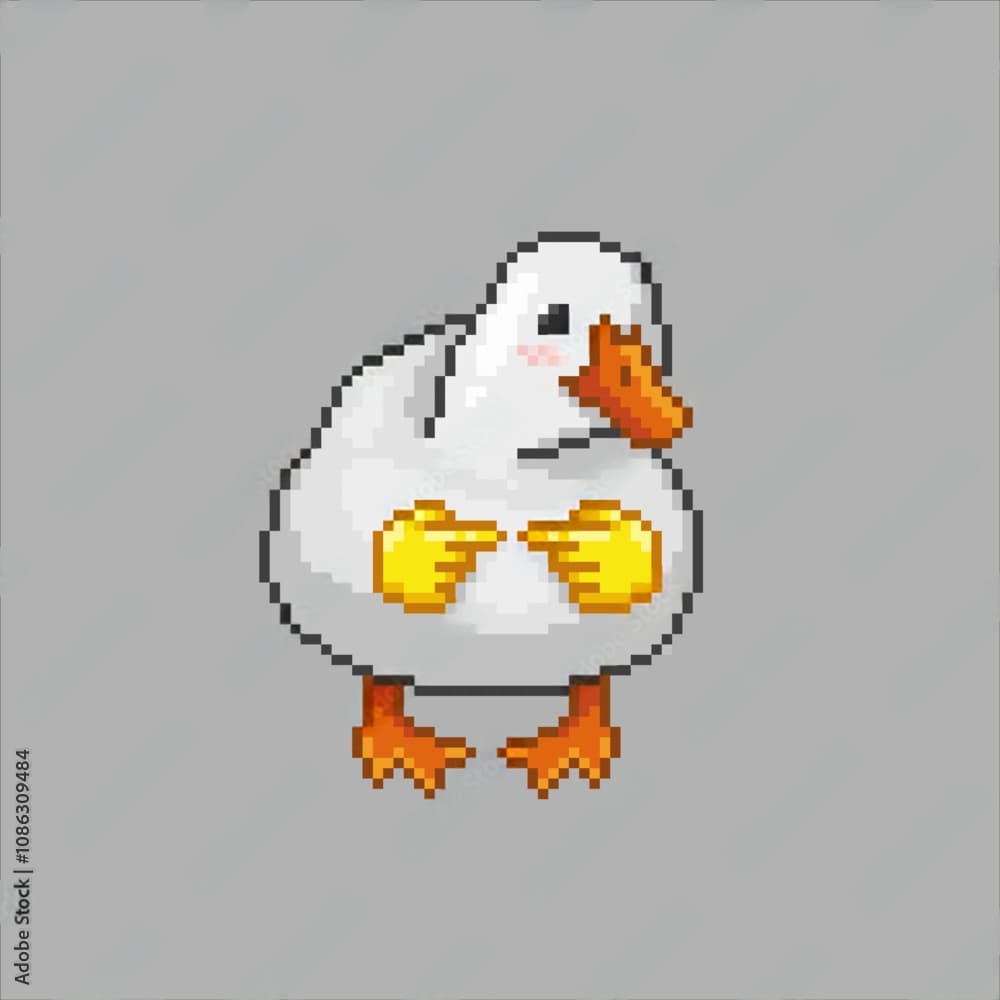
The Future of Jobs: Which Careers Will Survive the AI Revolution?
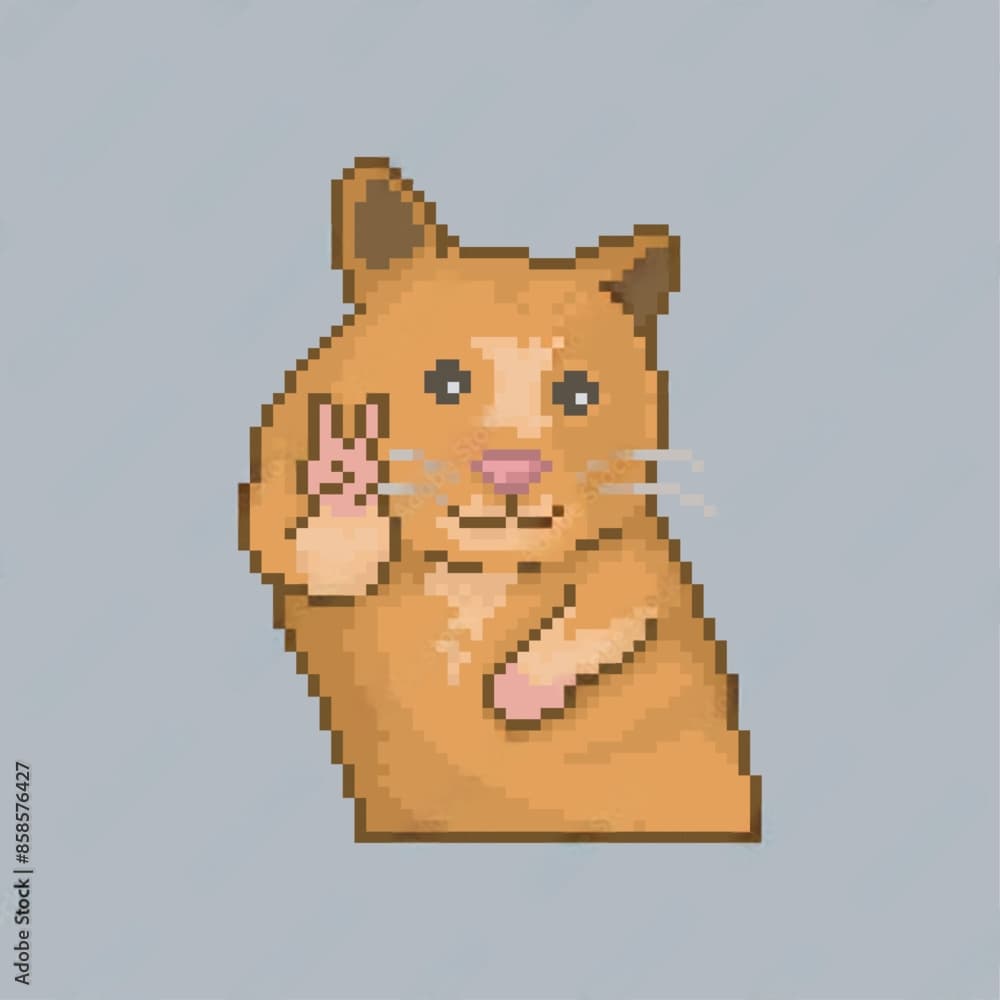
The Truth Behind Those Handcrafted Leather Bags and Watches: How AI, Actors, and Cheap Goods Are Fooling Shoppers Online
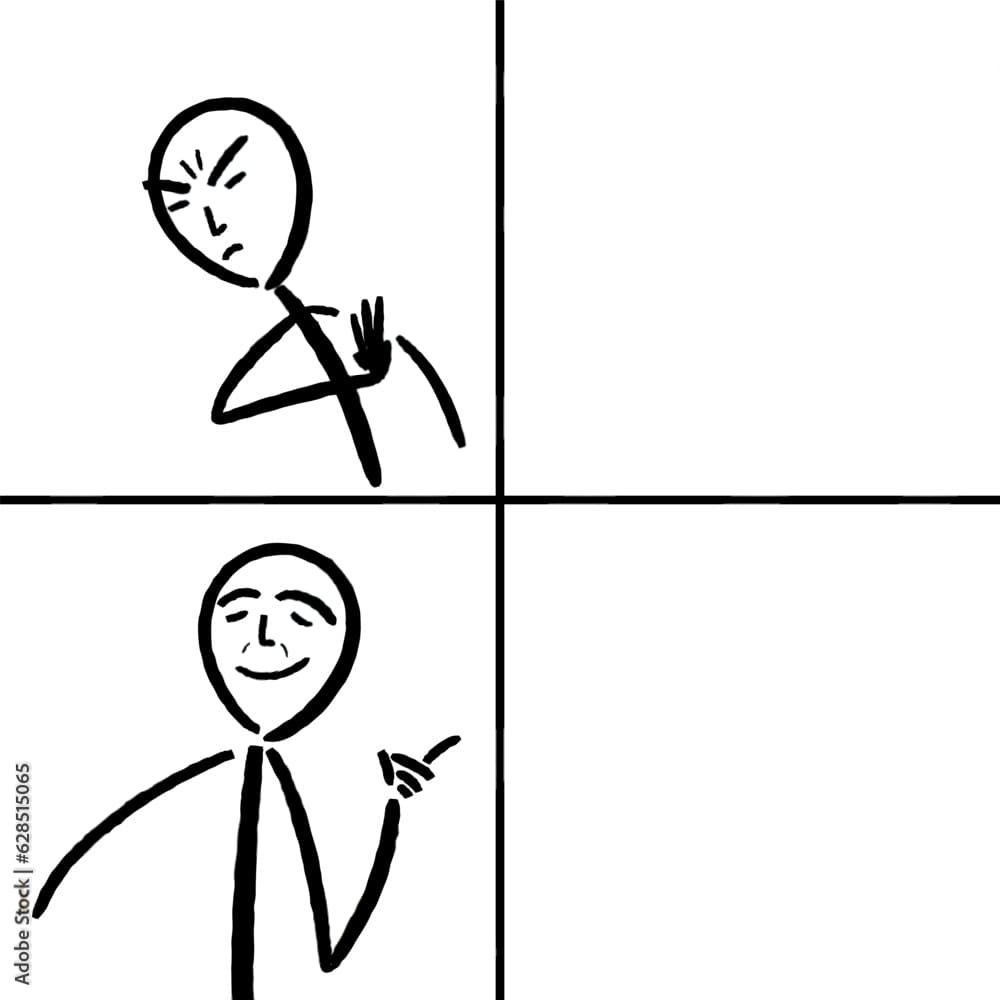
Will AI Replace Programmers? A Veteran Engineer on the Future of Software Jobs
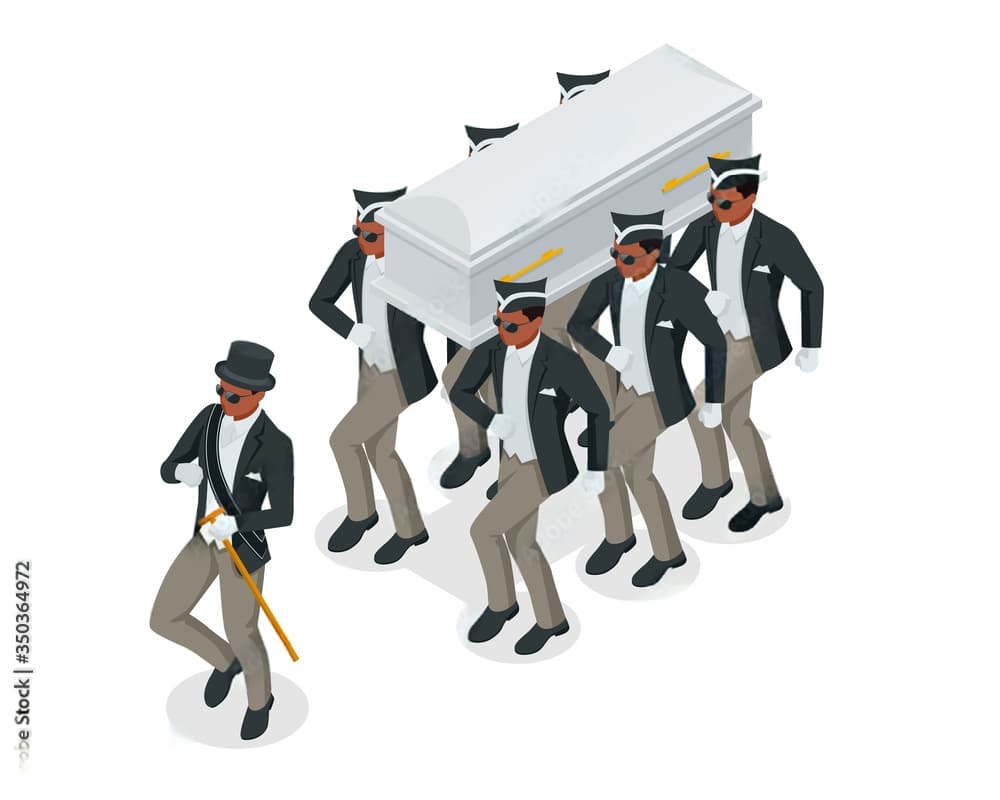
Inside the Secret World of Technical Interview Cheating: Tactics, Temptations, and Terrible Consequences
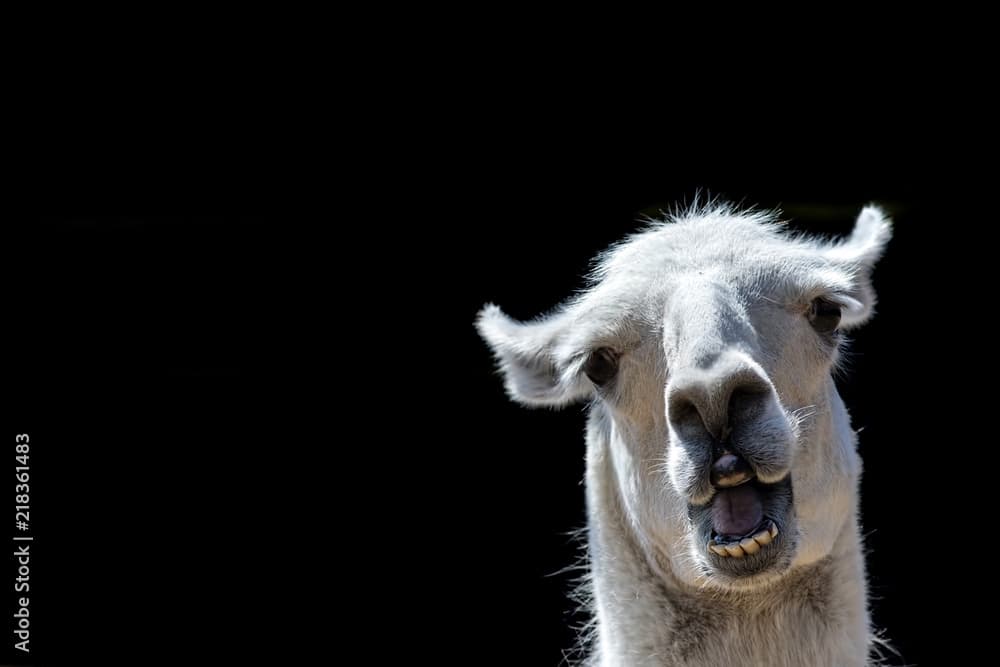
AI Agents Demystified: The Step-by-Step Guide for Non-Techies Using Real Life Examples
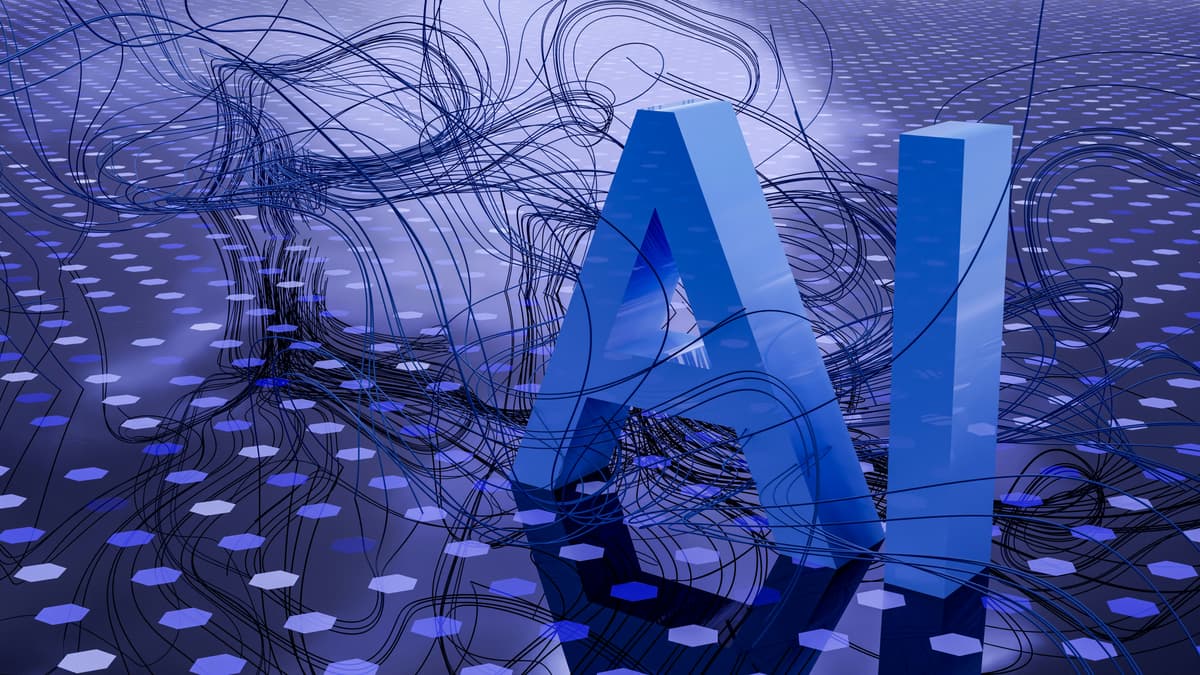
Is AI Making Us Dumber? Navigating the Cognitive Costs of Automation in the Knowledge Age
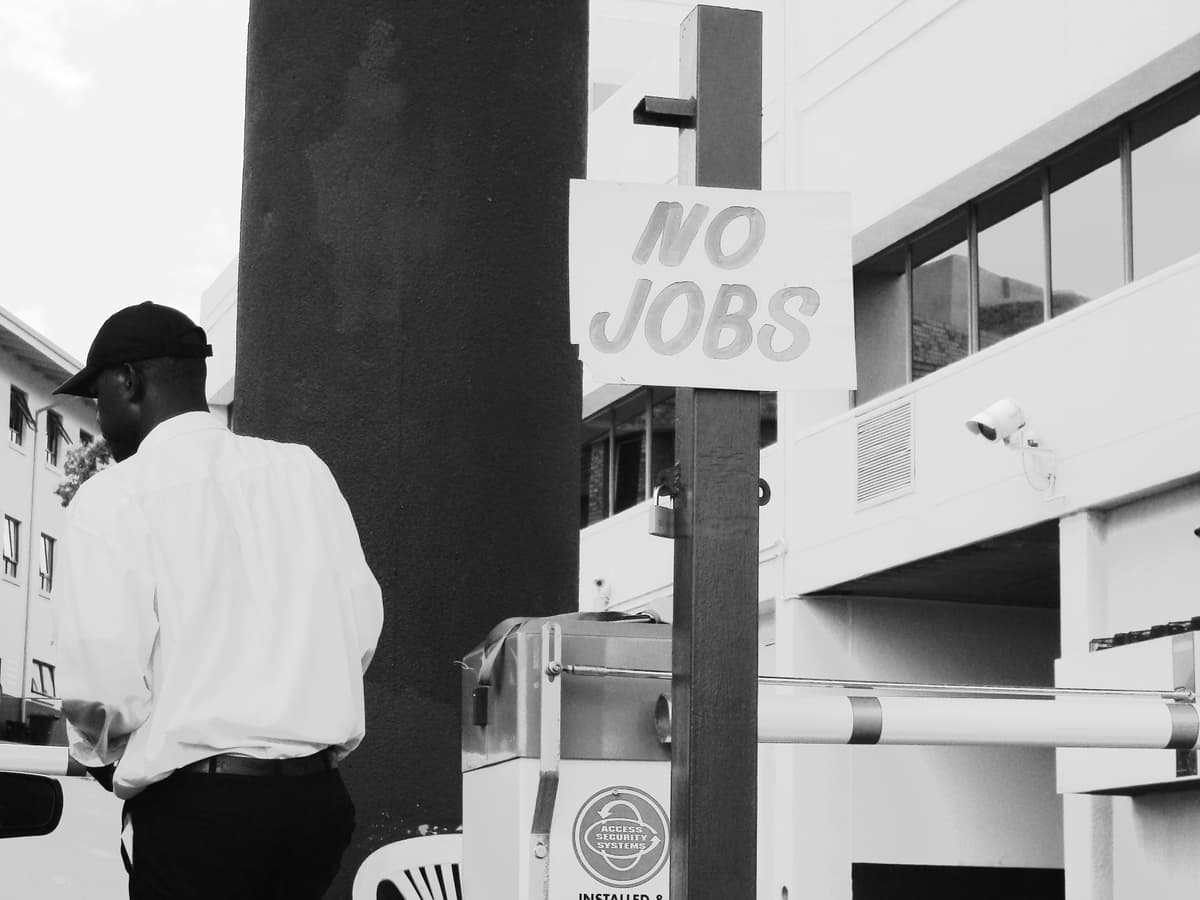
The Death of Coding: Why Chasing Tech Jobs Might Keep You Broke in the Age of AI and Bitcoin
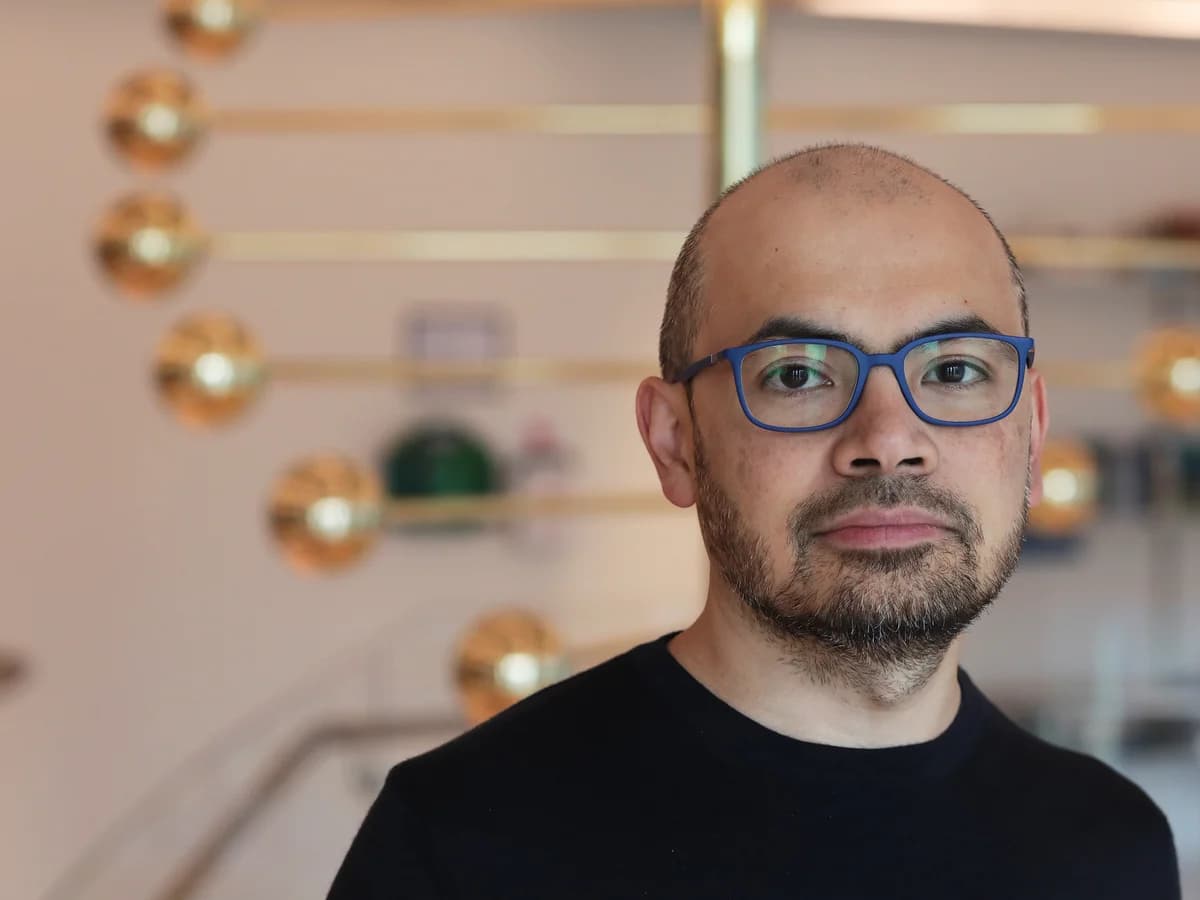
Beyond the Nobel: Demis Hassabis, DeepMind, and the Race Toward Superhuman AI
![The moment we stopped understanding AI [AlexNet]](/_next/image?url=https%3A%2F%2Fres.cloudinary.com%2Fdhgjhspsp%2Fimage%2Fupload%2Fv1746105277%2Fzuzana-ruttkay-1kslaBtXBk8-unsplash_ebqdgh.jpg&w=1200&q=75)
The moment we stopped understanding AI [AlexNet]
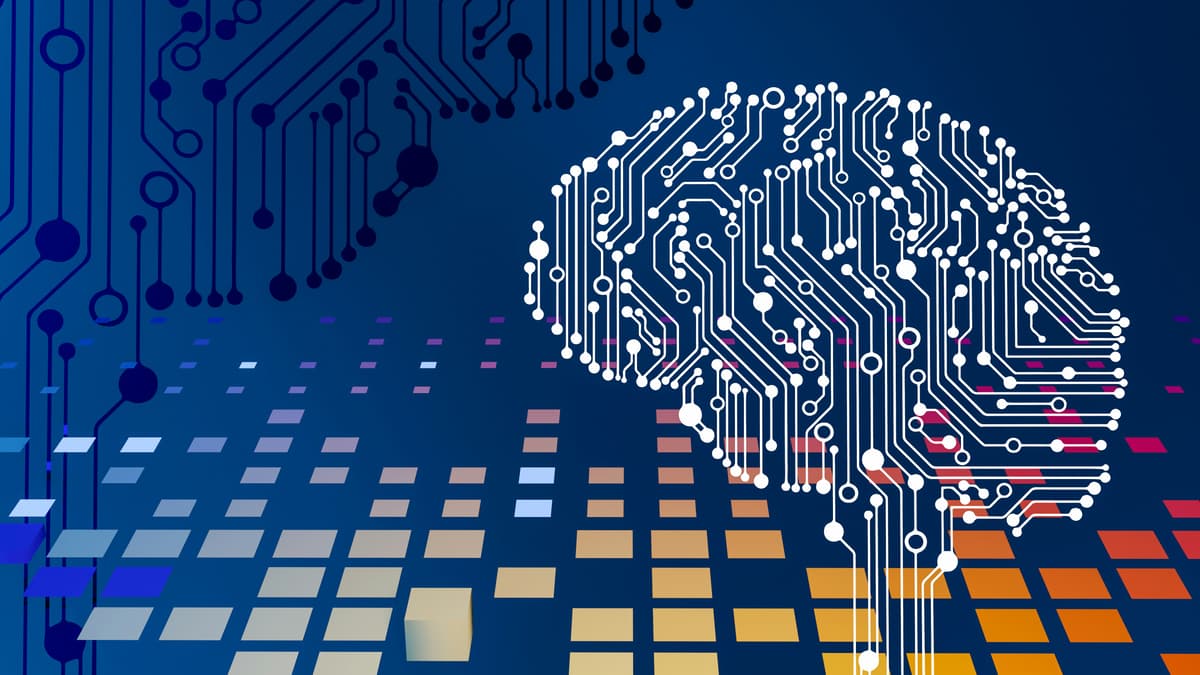